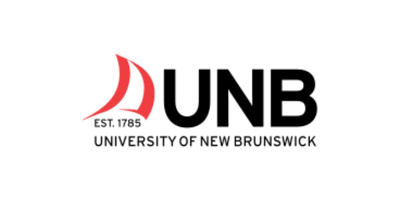
Integrated Multi-Trophic Aquaculture (IMTA) refers to managed, multi-species systems which recycle the byproducts of one aquatic species as nutritional inputs for another. These systems aim to improve environmental management, and increase harvest value through product diversification and recycling of nutrients. However, for these benefits to be realized, all the interacting components must be understood and carefully optimized. Dr. Gregor Reid, a senior research scientist with the University of New Brunswick, has used @RISK to determine the ideal ratios of kelps to absorb fish waste matter from a salmon IMTA system, as a means to better help aquaculturists improve the efficiency of seafood farming operations. A paper detailing this work appeared in the journal Aquaculture in May of 2013.
Addressing the Environmental Impact of Commercial Aquaculture of Salmon
Aquaculture has become a booming industry in many regions of the world. According to the World Bank, nearly two-thirds of the seafood we consume will be farm-raised in 2030. However, as with all forms of intensive food production, commercial aquaculture is not immune to the potential for environmental impacts. Modern intensive fish farms produce large amounts of nutrient waste, both as solid-organic (feces and waste feed), and dissolved (carbon dioxide, ammonia and phosphate). If a farm is poorly located with limited flushing, or nutrient loading exceeds environmental assimilative capacity, algal blooms (i.e. eutrophication) and low oxygen may result, impacting the localized environment. Since these nutrient forms are the same as natural inputs for shellfish that filter organic particulates, and for kelps that absorb soluble inorganic nutrients, this provides an ideal opportunity to mitigate fish waste, while augmenting nutritional inputs for other marketable culture species.
While the principle of growing multiple aquatic species in close contact has been done informally for thousands of years, “We’re now on a much bigger scale,” says Reid. “We’re now trying to apply the historical benefits of polyculture [the simultaneous cultivation of different species] to the typical large monocultures seen in aquaculture today, by connecting adjacent culture groups by nutrient transfer through water.”
The system Reid works on, located in the Quoddy region of the Bay of Fundy, Canada, involves farmed Atlantic salmon and two different species of kelp: winged kelp (Alaria esculena) and sugar kelp (Sacharina latissimi). Salmon are a valuable seafood species, while kelps are used in a broad array of consumer products. If grown in close proximity and in the right ratios, these two species can benefit each other, along with the environment. This extra nutrient availability can increase kelp growth, resulting in a more profitable harvest. In turn, with enough kelp, oxygen levels can be boosted and nutrient absorption can reduce the impact of fish waste on the surrounding aquatic environment.
However, finding the appropriate ratios of salmon to kelp to achieve these nutrient transfer benefits is tricky, thereby making the benefits of large-scale commercial IMTA operations difficult to execute. While researchers have previously attempted to determine appropriate ratios, “past recommendations have been in algae per square meter, which doesn’t really mean much to someone without a lot of experience in this field,” says Reid. His goal was to create a straightforward measure easily understandable for application to an IMTA operation. To do this, he created a ratio model that reports the weight ratio of harvested seaweeds required to sequester an equivalent soluble nutrient load per unit growth of fish. More specifically, for every x kg of salmon grown, y kg of kelp would need to be harvested, to remove the same amount of nutrients produced during the x kg growth of salmon.
Gathering the Inputs using @RISK’s Distribution Fitting Tool
Reid used a semi-stochastic approach for data that has high variability, such as nutrient loading estimates, in order to quantify uncertainty in the model.
Reid gathered inputs from three diverse sources: the commercial industry, academic literature, and field research. Rather than use single static values for the model inputs, Reid used stochastic distributions for several inputs with ranges of uncertainty, such as digestibility of salmon feed components (e.g. protein, fats, carbohydrates) and nutrient content of IMTA kelps. While known inputs or easily determined values, such as feed composition (listed on all feed bags) could be left as static inputs. Model inputs with sufficient empirical sample data were fit with the @RISK’s distribution fitting functions, testing 15 different theoretical distribution types, and using maximum likelihood estimators to rank the best fits.
Reid had to be careful when considering input data. “Often, you’re trying to fit a theoretical data distribution, but there are certain properties in a theoretical data distribution that won’t exist in real life,” he explains. “There are distributions that assume the tails go on forever, but that’s never going to happen. So you have to apply data filters on those to get more realistic results.”
Dr. Gregor Reid
Senior Research Scientist, University of New Brunswick
@RISK Helps Determine Proper Ratios of Kelps to Salmon for Commercial Aquaculture Farms
Reid used @RISK to run a simulation of 10,000 iterations using these inputs. The results gave a weight ratio model, with ratio estimations for each key nutrient, as well as oxygen supply potential for both species of kelp (see graphs).
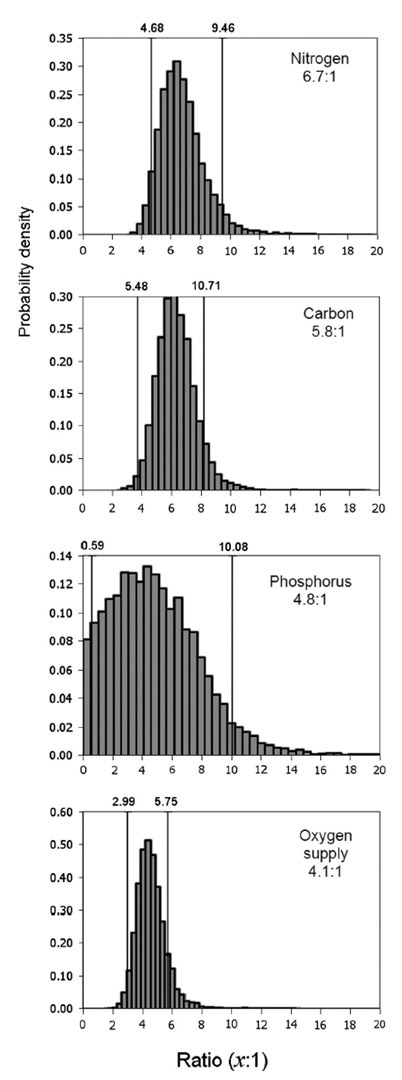
Figure 1: Weight ratios of the kelp, Alaria esculenta, required to sequester nutrients and supply dissolved oxygen per unit growth of Atlantic salmon (Salmo salar).
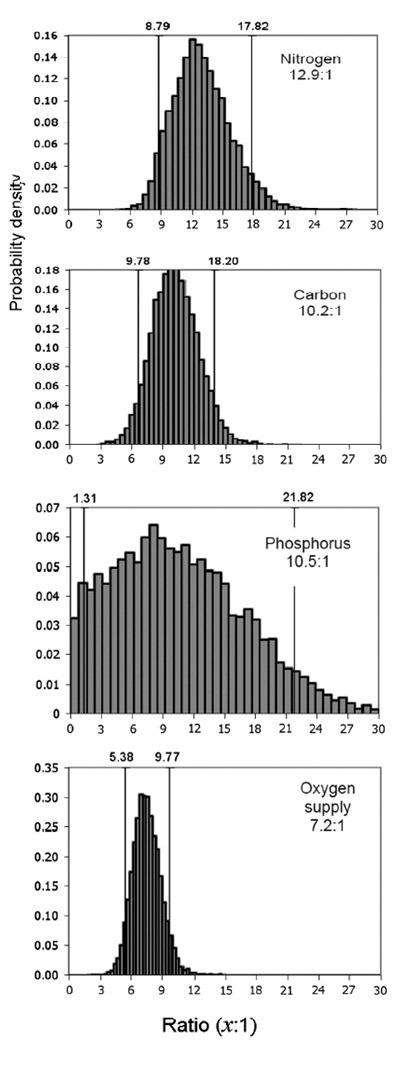
Figure 2: Weight ratios of the kelp, Saccharina latissima, required to sequester nutrients and supply dissolved oxygen per unit growth of Atlantic salmon (Salmo salar).
In order for a commercial-scale salmon farm to have all its fish waste fully absorbed by kelp the number of rafts of kelp would have to significantly outnumber the salmon pens, which is impractical for most North American operations where the space available for coastal fish-farming is highly limited and regulated. “It’s not going to be possible to do full dissolved nutrient recovery with seaweeds such as kelps in North America, unless the the number of fish being farmed on site is reduced to make room for other species,” says Reid. “It would be tough for commercial salmon-culture operators to do that, because presently there is a larger return for fish per unit space than other cultured species.”
However, Reid notes that “one-hundred percent nutrient sequestration doesn’t need to be the only successful endpoint in such systems. Removal of any nutrient portion has merit and this is presently occurring with kelp rafts deployed at the edges of some salmon farm lease areas.” He also says that smaller-scale farms may be in a better position to alter the balance of species groups, by raising a premium priced fish species, thereby enabling a financial return from smaller fish culture numbers.
Reid says that @RISK was instrumental in his study: “@RISK has been a great way to communicate uncertainty in complex systems, and its ease of use with Excel spreadsheets make it a highly intuitive and powerful tool.”